Types of loads on structures are crucial concepts in civil engineering and structural design. Structures are designed to support various types of loads and forces, which can act on them from different directions and with varying magnitudes. Understanding these loads is essential for designing safe and stable structures. In this comprehensive explanation, we’ll cover everything you need to know about the various types of loads on structures, including their definitions, characteristics, sources, and effects.
1. Introduction to Loads on Structures
Structures, whether they are buildings, bridges, or any other engineered systems, are subjected to various forces and loads throughout their lifetime. These loads can be categorized into two main groups: static loads and dynamic loads.
Static loads are those that do not change with time and are typically a result of gravity, such as dead loads and live loads. Dynamic loads, on the other hand, vary over time and are often caused by environmental factors like wind, snow, and seismic activity. Understanding the different types of loads and their effects is essential for designing structures that are safe, durable, and cost-effective. Fig. 1 below shows some examples of complex structures.
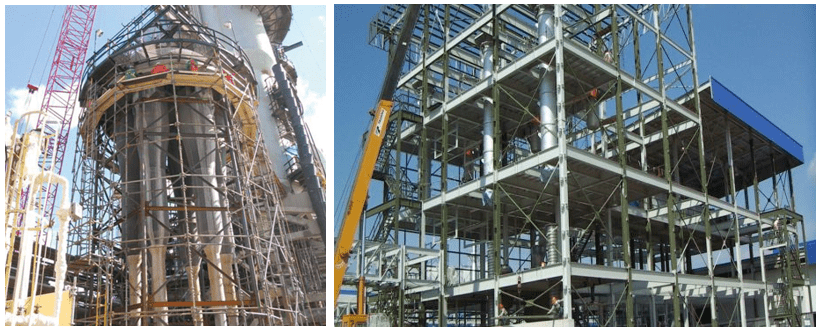
2. Dead Loads
Dead loads, also known as permanent or static loads, are the static forces that act on a structure due to its own weight and the weight of permanently attached fixtures and materials. These loads remain relatively constant and do not change with time.
Major Types of Dead loads include:
Self-weight of Materials: The weight of the structural elements themselves, such as beams, columns, walls, and floors. This is the most significant component of dead load and includes the weight of materials such as concrete, steel, wood, bricks, and roofing materials.
Permanent fixtures: These include items like HVAC systems, plumbing, electrical fixtures, and architectural elements that are permanently attached to the structure.
Finishes: Materials like floor finishes, wall coverings, and ceiling materials that are part of the building’s interior.
Permanent Partitions: Walls and partitions that cannot be moved are considered part of the dead load.
Architectural Components: Elements like exterior cladding, facades, and decorative features contribute to the dead load.
Earth Retaining Structures: In cases of retaining walls and embankments, the weight of the retained soil or fill is considered a dead load.
Miscellaneous Permanent Loads: These include items like fire protection systems, sprinkler systems, and built-in lighting fixtures.
Dead loads are typically the most predictable loads in structural design because they can be precisely calculated based on the known properties and dimensions of the materials used. Their main effects are:
Dead loads primarily act vertically downward.
- They provide stability to the structure by counteracting external forces.
- Engineers must account for dead loads when designing foundations, columns, and beams to ensure structural integrity.
3. Live Loads
Live loads, also known as transient loads or imposed loads, are dynamic forces that change over time and are caused by the presence and movement of people, furniture, equipment, and other temporary loads within a structure. Live loads can vary significantly depending on the purpose and occupancy of the structure.
Examples of live loads include:
Occupant loads: The weight of people inside a building, varies based on the building’s use and occupancy.
Furniture and equipment: Loads from items like desks, chairs, appliances, and machinery.
Storage loads: Loads from stored materials and equipment in warehouses or storage facilities.
Live loads are less predictable than dead loads because they depend on how the structure is used and can change over time. Engineers use building codes and standards to determine appropriate live load values for different types of structures and occupancies.
4. Wind Loads
Wind loads are dynamic forces exerted on a structure due to the movement of air. Wind loads can vary in magnitude and direction, making them a significant consideration in structural design, especially for tall buildings, bridges, and other exposed structures.
Key factors influencing wind loads include:
Wind speed: The local wind climate and the height of the structure determine wind speed. Higher wind speeds result in larger wind loads.
Wind direction: Wind loads can vary depending on the angle at which the wind strikes the structure. Engineers consider multiple wind directions to assess the structure’s overall stability.
Shape and exposure of the structure: The shape, height, and surface roughness of the structure can affect wind pressure distribution.
Terrain and Surroundings: The terrain around the structure, such as open fields or urban areas, can affect wind flow and pressure.
Building Components: Elements like canopies, antennas, and roof-mounted equipment can create additional wind loads due to their shape and location.
To calculate wind loads, engineers use wind load codes and standards, which provide equations and procedures for determining the forces and moments caused by wind on different parts of the structure. Wind loads are critical for ensuring a structure’s stability and safety, particularly in regions prone to strong winds.
Effects of Wind Loads:
- Wind loads can cause lateral movement and sway in a structure, which must be controlled to prevent discomfort or damage to the building.
- Engineers design structures to resist wind loads by specifying appropriate materials, reinforcements, and structural configurations.
5. Snow Loads
Snow loads are static forces exerted on a structure due to the weight of accumulated snow and ice. These loads are primarily relevant in regions with cold climates, where snowfall is common. The magnitude of snow loads depends on several factors, including:
Location: The geographic location and elevation determine the typical snowfall patterns and snow load requirements for a given area.
Roof shape: The slope and geometry of a roof can affect the accumulation of snow.
Snow density: The density of the snowpack, which can vary depending on weather conditions, impacts the snow load.
Duration of Snowfall: The longer the snow accumulates, the greater the snow load becomes.
Snow loads are particularly important for buildings and structures in regions with cold climates or mountainous terrain. Engineers use snow load data provided by local building codes to design roofs and supporting structures that can safely withstand the weight of snow and ice.
Effects of Snow Loads:
Snow loads can cause structural damage or even collapse if not properly accounted for in design and construction.
Engineers must calculate and consider snow loads when designing roofs and supporting structures in snow-prone areas.
6. Seismic Loads
Seismic loads, often referred to as earthquake loads, result from the ground motion caused by seismic events, such as earthquakes or volcanic eruptions. These loads are dynamic and can cause significant lateral and vertical movement and deformation in a structure. The intensity of seismic load varies based on the seismic activity of a region.
Key factors affecting seismic loads include:
Seismic hazard: The level of seismic activity in a region, as quantified by seismic hazard maps and geological studies.
Soil conditions: The type and properties of the soil or foundation materials can affect the way seismic waves propagate through the ground.
Building/Structure Height and Mass: Taller and heavier buildings tend to experience higher seismic loads
Building design response spectra: Engineers use response spectra to represent the relationship between ground motion and building response at various frequencies.
Building Materials and Construction: The choice of materials and construction methods can influence a structure’s ability to withstand seismic forces.
Designing for seismic loads is essential in earthquake-prone areas to ensure the safety and resilience of structures. Engineers use seismic design codes and standards to calculate the forces and displacements that a structure may experience during an earthquake and design the structure accordingly.
Effects of Seismic Loads:
- Seismic loads can cause structural deformation, cracking, and even collapse if not adequately addressed in design and construction.
- Engineers use seismic design codes and conduct seismic analyses to ensure buildings can withstand seismic events safely.
7. Thermal Loads
Thermal loads result from temperature variations and the resulting expansion and contraction of materials within a structure. These loads can affect the integrity and stability of a building’s components. Thermal loads can lead to structural deformations, stress, and potential damage if not properly accounted for.
Key factors related to thermal loads include:
Temperature fluctuations: Daily and seasonal temperature changes cause materials to expand and contract.
Thermal gradients: Temperature differences between different parts of a structure can induce stress and deformation.
Material properties: The coefficient of thermal expansion (CTE) of materials determines how much they expand or contract with temperature changes.
Restrained Conditions: Thermal loads can be more pronounced in structures with limited movement, such as bridges with fixed supports.
Engineers must account for thermal loads in structural design to prevent issues like thermal stress, distortion, and differential movement between structural elements. Expansion joints, flexible materials, and appropriate material selection can help mitigate thermal effects.
Effects of Thermal Loads:
- Thermal expansion and contraction can lead to structural movement, stress, and fatigue.
- Engineers design expansion joints, use appropriate materials, and consider thermal effects to mitigate the impact of thermal loads.
8. Foundation Settlement Loads (FSL)
Foundation settlement loads result from the gradual sinking or settlement of a structure’s foundation over time. Settlement can occur due to various factors, including soil consolidation, shrinkage, and differential settlement. Understanding and predicting settlement is crucial for maintaining a structure’s stability.
Factors Affecting Foundation Settlement Loads are
Soil Type: The type and properties of the soil beneath the foundation play a significant role in settlement behavior.
Foundation Design: The design and construction of the foundation, including its depth and type (e.g., shallow or deep foundations), can influence settlement.
Structural Load: The magnitude and distribution of structural loads on the foundation can affect settlement.
Water Table: The presence of a high water table can lead to soil saturation and increased settlement.
Effects of Foundation Settlement Loads:
Excessive settlement can lead to structural damage and compromise the safety of a building.
Engineers employ various techniques, such as proper foundation design, soil improvement, and monitoring, to mitigate settlement effects.
9. Hydrostatic Loads (HL)
Hydrostatic loads result from the pressure exerted by liquids, such as water, on submerged or partially submerged structures. These loads can act horizontally or vertically, depending on the orientation of the structure and the liquid’s depth. Hydrostatic loads are particularly relevant for structures like dams, reservoirs, and underwater tunnels.
Factors Affecting Hydrostatic Loads are
Liquid Density: The density of the liquid affects the magnitude of hydrostatic pressure.
Liquid Depth: The depth of the liquid above the structure determines the hydrostatic load’s intensity.
Shape and Orientation: The shape and orientation of the submerged portion of the structure influence the distribution of hydrostatic pressure.
Effects of Hydrostatic Loads:
- Hydrostatic pressure can cause structural deformation, leakage, or even failure if not properly accounted for.
- Engineers use principles of fluid mechanics to calculate hydrostatic loads and design structures accordingly.
10. Blast Loads (BL)
Blast loads result from explosive detonations and are a significant concern for structures in proximity to military facilities, industrial sites, or areas prone to terrorist attacks. Blast loads can exert both shockwave and debris impact forces on a structure, leading to severe damage if not adequately designed for.
Factors Affecting Blast Loads are
Blast Energy: The energy and size of the explosion determine the magnitude of the blast load.
Distance from the Explosion: Proximity to the explosion source significantly influences the blast’s intensity.
Building Vulnerability: The design and construction of the building, including its resistance to shockwaves and debris, affect its ability to withstand blast loads.
Effects of Blast Loads:
- Blast loads can cause structural damage, fragmentation, and collapse.
- Engineers employ blast-resistant design principles, materials, and protective measures to enhance a structure’s resilience to blast events.
11. Dynamic Loads (DL)
Dynamic loads are transient forces that result from dynamic events such as machinery operation, equipment impact, or vibration. These loads can cause structural vibrations, fatigue, and, in extreme cases, structural failure if not properly addressed.
Sources of Dynamic Loads are
Machinery Operation: The operation of heavy machinery, such as industrial equipment or rotating machinery, can induce dynamic loads on the structure.
Earthquakes and Seismic Activity: As previously discussed, seismic loads are dynamic in nature and can cause dynamic responses in structures.
Impact Loads: Impact from objects, such as falling debris or vehicular collision, can generate dynamic loads.
Effects of Dynamic Loads:
- Dynamic loads can lead to structural vibrations, which must be controlled to prevent discomfort or damage to the building’s occupants and contents.
- Engineers use dynamic analysis and design techniques to address dynamic loads and ensure structural safety.
12. Other Miscellaneous Loads
In addition to the main types of loads mentioned above, there are other miscellaneous loads that engineers need to consider in structural design. These loads are less common but can still impact a structure’s performance. Some examples include:
Crane loads: For industrial or construction facilities, engineers must consider the loads generated by cranes and heavy machinery.
Environmental loads: Loads from environmental factors such as ice, rain, hail, or sandstorms can affect certain types of structures.
Traffic loads: Bridges and highway structures must be designed to withstand the weight and motion of vehicles.
Tidal Loads: Buildings and structures near coastlines may experience tidal loads due to the rise and fall of tides.
Seiche Loads: Seiche loads result from the oscillation of water in closed or partially enclosed bodies of water, such as lakes and harbors.
Vibration loads: Vibrations from sources like machinery or nearby transportation can affect the structural integrity of buildings.
Construction Loads: During construction, temporary loads from equipment, materials, and construction activities can stress the structure.
13. Load Combinations
In structural design, it’s essential to account for the simultaneous effects of multiple loads, as structures are rarely subjected to just one type of load at a time. Load combinations are used to analyze the structure’s response to various loads occurring simultaneously or sequentially. The most common load combinations are based on building codes and standards and typically include:
Dead load + Live load: This combination accounts for the permanent weight of the structure and any live loads it may experience during its lifespan.
Dead load + Wind load: It considers both the weight of the structure and the wind forces it might encounter.
Dead load + Snow load: This combination is crucial in cold climates and regions prone to heavy snowfall.
Dead load + Seismic load: Particularly important in earthquake-prone areas, this combination assesses the structure’s response to gravity and seismic forces.
Each load combination has specific load factors that reflect the relative importance of the different loads. Engineers use these load combinations to ensure that the structure can safely withstand all anticipated loads without exceeding its design limits.
14. Load Paths and Load Distribution
In addition to understanding the types of loads, engineers must consider how these loads are transmitted through a structure. Load paths and load distribution are critical aspects of structural design. A load path refers to the route that forces and loads follow as they travel through a structure, from their point of origin to the foundation and ultimately to the ground. Proper load paths are essential to ensure that forces are efficiently transferred and distributed to prevent structural failure.
Load distribution involves ensuring that the loads are appropriately distributed among the structural elements, such as beams, columns, walls, and foundations. Engineers use structural analysis techniques to determine the internal forces and stresses within each element and ensure that they remain within safe limits. Proper load distribution ensures that no single element is overloaded, which could lead to structural failure.
15. Structural Analysis and Design
The analysis and design of structures involve complex engineering calculations and considerations. Structural engineers use various methods and tools to ensure that a structure meets safety, stability, and performance requirements. Some key steps in structural analysis and design include:
Load calculations: Engineers calculate the loads acting on the structure based on their type, magnitude, and location.
Structural modeling: Using computer-aided design (CAD) software and finite element analysis (FEA), engineers create detailed models of the structure to simulate its behavior under different loads.
Stress analysis: Engineers assess how forces and loads affect the internal stresses and deformations of structural elements, ensuring they stay within acceptable limits.
Material selection: The choice of materials, such as concrete, steel, wood, or composites, is critical to meet design requirements and withstand the anticipated loads.
Cross-section design: Engineers determine the size and shape of structural elements (e.g., beams, columns) to safely carry the loads.
Foundation design: Foundation systems must be designed to support the structure and transfer loads to the ground efficiently.
Reinforcement and connections: Engineers specify reinforcement materials and design connections between structural elements to ensure integrity and safety.
Code compliance: Designs must adhere to local building codes and standards to meet safety and regulatory requirements.
Load testing and analysis: In some cases, load testing of prototypes or existing structures may be necessary to validate design assumptions and ensure safety.
Structural analysis and design require a deep understanding of structural mechanics, materials science, and engineering principles. Engineers must also consider factors such as aesthetics, constructability, and cost-effectiveness in their designs.
16. Conclusion
In summary, understanding the various types of loads on structures is fundamental to the field of civil engineering and structural design. Engineers must consider dead loads, live loads, wind loads, snow loads, seismic loads, thermal loads, foundation loads, and other miscellaneous loads when designing safe and resilient structures. Proper load calculations, load combinations, load paths, and load distribution are essential for ensuring structural integrity and safety.
The complex interplay between these loads and the structural elements requires advanced analysis and design techniques, often aided by computer simulations and modeling. Engineers must also adhere to building codes and standards to meet regulatory requirements and ensure the safety of occupants and the longevity of the structure.
In the ever-evolving field of structural engineering, continued research and innovation are essential to improve our understanding of loads on structures and develop more efficient, sustainable, and resilient structural designs. Engineers play a crucial role in shaping the built environment, and their expertise in load analysis and design is vital for constructing safe and enduring structures that enhance the quality of life for people worldwide.